Advanced heart failure is a challenging condition to treat and has long been associated with significant morbidity for afflicted patients.1,2 Guideline-directed medical therapy has improved outcomes, helped prevent sudden death, and improved symptoms for these patients. But treatment of the most advanced forms of end-stage of heart failure requires one of two surgical treatment modalities: implantation of ventricular assist devices or orthotopic heart transplantation. First initiated in 1966 and 1967, respectively, the use of ventricular assist devices and heart transplantation have matured, evolved, and notably improved since their initial introductions. These two surgical treatments are intimately intertwined, often serving as complementary or stepwise therapies in the longitudinal management of end-stage heart failure.
New technological developments, improvements in surgical techniques, and the evolution of nationwide policies have driven and continue to drive both fields forward. Here we provide a historical perspective on the development of durable ventricular assistance and orthotopic heart transplantation, along with a focus on the recent evolutions, changes, and controversies in these fields that have significantly influenced practice.
Historical Perspective
The origins of ventricular assistance – and much of the early history of orthotopic heart transplantation – is inextricably linked to Houston, Texas. Initial exploration into the field of left ventricular assistance by Michael DeBakey, William Kolff, Tetsuzo Akutsu and Domingo Liotta at Baylor College of Medicine in the early 1960s focused on the development of a left ventricular assist device (LVAD), which would support the left ventricle temporarily to allow for recovery in patients who were experiencing significant difficulty weaning from cardiopulmonary bypass. The concept of ventricular rest to allow for recovery was developed in this era, and stemmed from the clinical observation that patients who had difficulty weaning cardiopulmonary bypass who were provided a more prolonged wean could occasionally recover.3 These observations stimulated future research into ventricular recovery, and culminated in the first successful use of what we would now call a bridge-to-recovery LVAD in September 1966 (Figure 1).
The motivation and these advancements in surgical support of ventricular function were paralleled by important advancements towards the successful implementation of heart transplantation. Pioneering canine research by Norman Shumway and Richard Lower at Stanford University on the successful use of hypothermia and the development of the orthotopic technique paved the way for human transplantation.4–6
Although much of the pioneering work on cardiac transplantation occurred in the US, the lack of clear ethical and legal guidelines outlining the definition of death in beating heart donation presented a significant obstacle for implementation in humans. However, in South Africa, opinions about the ethical nature of what would now be considered donation after neurological death were considerably more favorable at the time, thus cracking open the door of human-to-human cardiac transplantation just enough for an ambitious surgeon to walk through.7,8 Christiaan Barnard performed the first successful cardiac transplantation in Cape Town, South Africa in December 1967.9
Barnard achieved technical success, which was subsequently demonstrated reproducible in Denton Cooley’s numerous early heart transplants in Houston. But early transplantation was plagued with difficulty related to azathioprine-based immunosuppression and associated overwhelming sepsis. Prior to the introduction of cyclosporine and the eventual development of comprehensive and safe immunosuppression for heart transplantation, significant doubts continued to exist on the longevity of cardiac grafts, providing incentive for the further development of durable ventricular assist devices.10 Even today, the long-term consequences of chronic immunosuppression are not trivial and some patients – even when eligible and offered a transplant – may elect to continue with an LVAD.
Development of left ventricular assistance over the subsequent decades initially focused on pulsatile pumps, although these devices were limited by their generally large size that precluded internal implantation in many patients and by the inevitable fatigue and critical failure of the flexing membranes that provided their pulsatile flow. These design challenges prompted development of more reliable continuous flow LVADs – devices that have become the predominant focus of the field since their introduction.
Although improved reliability and implantability of continuous-flow pumps eventually resulted in their more widespread use, continuous-flow LVADs were not without their own negative sequalae. Complications of red blood cell lysis from the pump speeds required to achieve flow, arteriovenous malformations as a result of continuous flow physiology, blood pressure lability related to baroreceptor dysregulation in the setting of decreased pulsatility, and device infection related to externalized LVAD drive lines are notable challenges. Significant improvements in design have mitigated many of these concerns, and continuous flow left ventricular assistance in the modern era has been established as a reliable therapy for alleviating heart failure symptoms and providing both quantity and quality of life for patients. The interplay between mechanical ventricular assistance and heart transplantation remains tight in the modern era, much as it was during its inception, and an understanding of the evolution of these surgical treatments remains critical to providing the best care for our heart failure patients.
Concomitant Procedures in Left Ventricular Assistance
Guidelines for the consideration for orthotopic heart transplantation or LVAD have been clearly outlined by international societies, and these guidelines form the foundation for evaluation of advanced heart failure patients at the authors’ institutions.11 As we expand the candidacy for patients receiving LVADs, it is inevitable that some patients with severe left ventricular dysfunction will also show concomitant cardiac pathologies that could be addressed at the time of LVAD implantation. A central question in considering these individuals as candidates for LVAD is the impact of addressing these pathologies concurrently with implantation of their durable LVAD.
A number of investigators have evaluated the impact of concurrent cardiac procedures on morbidity and mortality after LVAD implantation.12,13 Pal and colleagues investigated the impact of concomitant repairs on individuals undergoing implantation of a HeartMate II (HMII; Abbott) LVAD over a 2-year period. Concomitant coronary artery bypass grafting, intracardiac repair of a patent foramen ovale, or repair of the tricuspid or mitral valve were not shown to increase 30-day mortality for these patients.12 Patients who underwent an aortic valve procedure at the time of LVAD implantation were found to have a significantly increased 30-day mortality at 25% compared with 5.8% mortality for isolated HMII implantation.12 This work has been validated by later studies with respect to tricuspid valvular repair and closure of patent foramen ovale with long-term follow-up to 2 years, although the observed increased mortality associated with aortic procedures has been debated.13
Some studies have demonstrated an increased risk with aortic procedures. However, it is important to note that aortic insufficiency that is moderate or greater on preoperative echocardiography may progress following LVAD implantation, creating a short recirculation loop that will decrease LVAD efficiency and worsen heart failure. As a result, aortic insufficiency should be treated aggressively.14,15 Multiple techniques exist for treatment of aortic insufficiency, ranging from a simple suture closure of the valve to traditional aortic valve replacement.16 Importantly, mechanical aortic valves increase the risk for embolic events in these patients, and should be avoided or explanted at time of LVAD implantation. Studies have suggested that aortic valve replacement may be superior to suture closure of the aortic valve in these patients. However, data have been conflicting, and aortic valve replacement undoubtedly increased aortic cross clamp time and ischemic time for these already dysfunctional ventricles.17,18 Suture closure of the aortic valve (or patch closure) is not without significant risk. Although there are immediate perioperative benefits to suture closure, permanent closure of the aortic valve will make the patient entirely LVAD-dependent for forward flow, and could result in sudden death in the setting of LVAD malfunction or failure.
Given the ever-expanding role of transcatheter aortic valve replacement (TAVR), the question arises whether TAVR may have a role in treating aortic insufficiency in patients receiving an LVAD. This is of particular interest in the setting of the late development of aortic insufficiency, which would require reoperative surgery with significant associated risk. TAVR has generally been reserved for aortic stenosis due to the calcific nature of the disease, which affords annular stability against the expanded stent. Despite this, TAVR has been successfully used in insufficient aortic valves, both with the self-expanding CoreValve (Medtronic), as well as the SAPIEN-3 (Edwards) balloon-expandable valve.19–21 One technique for employing TAVR in the setting of prior LVAD uses a combined deployment of a CoreValve into the aortic annulus, followed by valve-in-valve SAPIEN-3 deployment with balloon expansion into the reinforced annulus to prevent paravalvular leak.22 Although limited data exist with this technique, TAVR provides an interesting alternative to other endovascular techniques for treatment of late aortic insufficiency such as aortic valve closure with an Amplatzer Occluder (Abbott), and therefore would not create a potentially fatal condition in the event of LVAD mechanical failure.
The demonstration of the safety of concomitant procedures – particularly right heart procedures – during LVAD implantation is a significant finding, especially as we aim to improve the long-term function of the right ventricle after LVAD implantation. In their 2014 study, Milano et al. built upon prior work by again demonstrating the safety of concomitant valvular procedures by demonstrating comparable survival at 1 year following implantation of the HeartWare centrifugal-flow ventricular assist device system (HVAD; Medtronic) for both those patients who underwent additional procedures and those who did not. However, importantly, the study demonstrated that patients with severe preoperative tricuspid regurgitation were significantly more likely to have late right ventricular failure following HVAD implantation when compared with those who underwent concomitant repair at the time of HVAD implantation.23 Given the safety of concomitant repair, and its impact on right ventricular functional preservation, tricuspid valvular repair at the time of LVAD implantation has become well established.
Minimally Invasive Approaches to LVAD Implantation
A great deal of focus in the advancement of outcomes related to LVAD implantation has rightfully been given to the development of new iterations of assist devices. However, as the field has matured, significant advancements in the technical approach towards implantation have occurred as well. Traditionally, LVADs have been placed via a full median sternotomy with assistance of centrally cannulated cardiopulmonary bypass. With the introduction of new, smaller LVAD pumps, such as the HVAD and HeartMate 3 (HM3, Abbott), less invasive approaches to LVAD implantation have been developed.
One notable advancement in technique has been the introduction of a minimally invasive, or sternotomy-sparing approach to LVAD implantation. Performed through the use of femoral cannulation for cardiopulmonary bypass, the apex of the left ventricle is approached through a left sided thoracotomy or subcostal incision to allow for left ventricular apical coring and implantation of the inflow portion of the LVAD (Figure 2).24 At the authors’ institution, the precise location of this limited thoracotomy is determined by intraoperative transthoracic ultrasound. The outflow graft is then tunneled through the pericardium and exits via either a right anterior thoracotomy or via an upper hemisternotomy to allow for access and grafting to the ascending aorta with assistance of a partial occlusion clamp. Careful attention to outflow graft placement location and angulation is critical to avoid kinking or compression of the RV.
Outcomes following this approach to LVAD insertion have been evaluated by several authors and have been safe and reproducible.25–27 These early studies culminated in a multicenter clinical trial for the HVAD, the LATERAL trial.28 Having enrolled patients from 26 centers across the US and Canada, the LATERAL trial evaluated outcomes up to 180 days following HVAD implantation via thoracotomy and compared with historical sternotomy-based data. Patients who underwent thoracotomy experienced greater survival at 180 days (88.1% versus 77.5%), shorter mean hospital stay (18 versus 36.1 days), and significantly reduced bleeding requiring reoperation compared with sternotomy-based approaches at 30-days, 6 months and 1 year following implantation.25,28
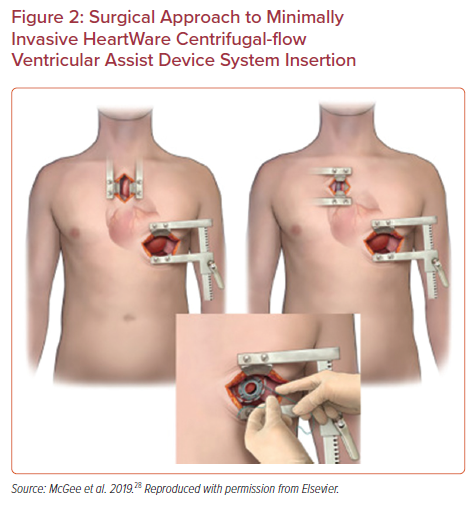
Potential benefits observed with a thoracotomy-based approach are the potential for the preservation of right ventricular function, as well as preservation of renal function postoperatively.29,30 Postoperative right ventricular failure has remained a significant cause for morbidity and is the single greatest risk factor for early mortality following LVAD implantation.31 By quantitative and qualitative assessment of perioperative right ventricular function in these patients, lateral thoracotomy-based LVAD implantation has been shown to significantly reduce right ventricular dysfunction as evident by pulmonary artery pulsatility index, right ventricular stroke work index, and echocardiographic assessment. These results persisted when evaluating those at high risk for right ventricular dysfunction, as evident by a EUROMACS-RHF score >4.29
The etiology behind preservation of right ventricular function through a thoracotomy-based approach is thought to be multifactorial. Reduced manipulation of the heart, in addition to preservation of the native pericardial attachments and restraint over the right ventricle may result in less perturbed right ventricle following implantation.32,33 Additionally, the reduced incidence of bleeding requiring exploration suggest less postoperative blood product resuscitation is required in these individuals, resulting in less resuscitation-based right ventricular overload and subsequent dysfunction.
The recent withdrawal of the HVAD from the market has created a potential void for devices with FDA approval for non-sternotomy implantation; however, the groundwork laid by the LATERAL trial and HVAD experience has established non-sternotomy approaches as safe and effective. Ultimately this experience has allowed for other devices to move into this market, and this void has been filled by the HM3, which has been approved for non-sternotomy implantation by the FDA.

United Network for Organ Sharing Allocation System Changes
One of the most wide-reaching and impactful recent changes in both the fields of mechanical ventricular support as well as orthotopic heart transplantation in the US has been the October 18, 2018 modification to the United Network for Organ Sharing allocation system for cardiac allograft donation. These changes aimed to reduce mortality for patients on the cardiac transplantation waiting list by prioritizing the sickest individuals for transplantation. The previous three-tier system for assessing priority for transplantation was deconstructed into six discrete categories (with ‘status 7’ reserved for temporary inactivation). This reorganization increased granularity in classifying the status of patients at the highest level of priority by separating the highest priority group from the previous allocation system into three distinct categories (Table 1). These changes significantly reduce competition amongst the sickest individuals listed for heart transplantation, and patients with well-functioning LVADs are no longer prioritized to the same degree.
Early evidence has clearly shown that the changes have achieved the desired effect of reducing transplant wait times for the sickest individuals listed, with average transplant wait times reduced from 35 days for status 1a patients under the pre-2018 system to 15 days for status 1 or 2 patients since the allocation system change.34,35 Additionally, the updated allocation system resulted in improved 180-day survival on the wait list (96.1% versus 95.0%). However, this has been associated with reduced 180-day post-transplant survival (77.9 versus 93.4%, p<0.0001) in early studies evaluating the outcomes post-policy change, as the hemodynamic instability of patients prioritized for transplantation has increased, as expected.36
In response to these allocation system changes, the use of temporary mechanical circulatory support (MCS) has significantly increased in the pre-transplantation setting, with 41% of patients currently undergoing transplantation from a temporary MCS device compared with 10% of patients prior to the change.35,36 The allocation system change has fundamentally changed the paradigm for the clinical treatment of acute decompensated heart failure for those patients who are candidates for heart transplantation. The previous conventional pathway for potential heart transplant candidates in acute decompensated heart failure typically consisted of inpatient stabilization followed by LVAD implantation. Patients subsequently underwent outpatient evaluation and listing for heart transplantation (Figure 3). Instead, inpatient stabilization for acute decompensated heart failure, often with the use of temporary MCS in conjunction with an expedited evaluation for heart transplantation has been adopted as a more viable pathway for transplantation in the current era.
Early data have confirmed these practice changes, showing a significantly reduced proportion of patients currently undergoing transplantation with a durable LVAD in place (42 versus 23%), an increased use of intra-aortic balloon pump prior to transplantation (45% versus 3%), and increased preoperative length of stay prior to transplantation (30% inpatient status versus 92%).35,36 Interestingly, these changes appear to be specific to the US. Evaluation the use of pre-transplant temporary MCS at US-designated transplant centers compared with Canadian centers has shown this increased use of support devices has been isolated to US-based centers, suggesting the change in organ allocation policy is driving the clinical behavior rather than a broad, physician-led adoption of temporary MCS prior to transplantation.37
These changes have also significantly impacted the approach to LVAD implantation. Prior to the 2018 allocation system change, destination therapy (DT) LVAD constituted a common, although not overwhelming, proportion of those patients receiving a durable LVAD when compared with the bridge-to-transplant (BTT) LVAD designation. However, following the allocation system change, DT LVAD indications at the time of implantation rose significantly, from 48–54% in the prior era to 70% following the policy change.38 As noted earlier, given the demotion of LVAD recipients to status 4 for heart transplantation, and the reduced complication profile of modern LVADs that might increase an individual’s wait list status, a shift in the intention at the time of implantation is an expected change. In many ways, the allocation system changes are reactive to and reflective of success in the field of durable mechanical circulatory support. With steady improvements in long-term survival and morbidity profile of patients maintained on LVADs, the urgency to transition to heart transplantation has been reduced.
The impact of policy change on practice behaviors will continue to be substantial, and recent changes in the Centers for Medicare and Medicaid Services (CMS) National Coverage Determinants are poised to impact the field further. In the updated policy of December 1, 2020, the CMS made significant changes to requirements for reimbursement for durable left ventricular assist devices by removing the prior therapeutic intent-to-treat criteria of BTT and DT for LVADs.39 Specifically, this policy change removes the reimbursement requirement that patients receiving and LVAD be active on the waitlist maintained by the Organ Procurement and Transplantation Network, and removes the requirement that an implanting institution receive permission from a Medicare-approved transplant center prior to LVAD implantation if that center is not itself a heart transplant center. The decision further extends the evidence-based patient selection criteria, previously reserved for DT LVADs, to all LVAD recipients. These changes, in effect, eliminate the practical requirement for a transplant evaluation prior to LVAD implantation. This effectively uncouples the heart transplant and LVAD centers, and opens the door for non-transplant centers to establish LVAD programs without the need for BTT or DT determinations.
These changes to CMS reimbursement guidelines reflect the changing landscape of LVAD therapy, and, importantly, reflect what has been shown to be a somewhat arbitrary differentiation BTT and DT designations. Advancements in LVAD technology and improved outcomes, specifically highlighted in the MOMENTUM 3 trial with respect to the HM3 LVAD, have demonstrated that in the current era, preoperative designation as BTT or DT has been unreliable. In their 2020 secondary analysis of the MOMENTUM 3 trial, Goldstein et al. observed significant crossover between BTT and DT therapeutic categories established at the time of HMII or HM3 implantation during a 2-year follow up period. Specifically, they observed a 13.5% orthotopic heart transplantation rate for patients initially deemed transplant ineligible by a DT categorization. They further demonstrated that 43% of patients categorized as BTT remained on their LVAD at the 2-year follow-up timepoint.40 The authors concluded that the use of categorizations based on patients’ current or future transplantation candidacy should be abandoned, and that adopting an implant-first strategy may improve survival and quality of life in these individuals.
Frailty in Patient Selection for LVAD and Transplantation
Frailty is complex syndrome associated with biological rather than chronological aging in patients, broadly characterized by progressive decline in functional reserves across multiple organ systems. Although multiple measures have been used, frailty is often measured using five clinically-measured domains of grip strength, exhaustion, unintended weight loss, slow gait speed, and low physical activity.41 Postoperative complications have been shown to be associated with markers of frailty, suggesting this is an important point of consideration prior to undergoing LVAD implantation or heart transplantation.42,43 Clinically frail patients have been shown to have increased time to extubation, increased hospital length of stay, and increased long-term mortality following LVAD implantation compared with non-frail individuals.44 Importantly, nevertheless, frailty appears to have little effect on short term mortality, and appears to have some reversibility with nearly half of patients having significant improvements in frailty indices after 3–6 months of LVAD support.42,44 Frailty in the setting of advanced heart failure should be conceptualized as frailty related to two separate conditions: LVAD-responsive frailty, which may improve with ventricular assistance, and generalized, or LVAD-independent frailty, which may persist despite LVAD implantation (Figure 4).
Similar to patients receiving LVADs, orthotopic heart transplant recipients who demonstrate preoperative frailty have demonstrated significant increases in a variety of postoperative complications. Frail individuals have demonstrated significantly increased rates of post-transplant length of stay, stroke, renal failure and mortality when compared to their non-frail counterparts at follow up extending up to 10 years post-transplant.45 The impact of frailty mechanistically on influencing outcomes following both LVAD and orthotopic heart transplantation is likely multifactorial. However, those individuals who exhibit frailty prior to major surgical procedures likely exhibit a significant depletion in physiological reserve resulting in a cumulative decline in the function of all physiologic systems. This depleted reserve and decline places them at unique risk for a lack of resiliency against stressors. The dramatic hemodynamic, immunologic, and inflammatory stressors associated with LVAD implantation and transplantation may exhaust this reserve, resulting in clinical decline.
Conclusion
Continued progress in both cardiac transplantation and LVAD have afforded patients with advanced, end-stage heart failure outstanding outcomes. These therapies have evolved in parallel and the future for both transplantation and LVADs remains bright. LVADs will continue to challenge the long-term success of transplantation, and this healthy competition is only expected to increase in intensity as the next generation of LVADs will be completely implantable and fully magnetically levitated. The patient-derived benefit and acceptance of a totally implantable LVAD will has the potential to be truly transformative.
Transplantation, while mature, also has tremendous potential for growth. Cellular-based therapies and the possibility of genetically modifying xenografts to an individual’s cell type (and therefore improving graft tolerance) have long been the holy grail in heart transplantation, and hold promise for alleviating the problem of donor heart scarcity. The community of providers who care for patients with advanced heart failure should remain poised to appropriately evaluate and test these new devices and potential therapies for the ever-growing patient population with advanced heart failure.