Cardiogenic shock (CS) remains a deadly disease entity, challenging patients, caregivers, and communities across the globe.1 The spectral and time-sensitive nature of CS is compromised by clinical heterogeneity and access to an interdisciplinary health system structure capable of delivering disease-specific diagnostic and therapeutic services.2 Clinical trials and registry-based studies have historically enrolled patients with CS that is refractory to fluid resuscitation and/or requires vasoactive agents to improve systolic blood pressure ≥90 mmHg. CS was defined by the presence of systemic hypoperfusion and tissue hypoxia from systolic blood pressure <90 mmHg for ≥30 minutes in the setting of severe myocardial dysfunction. However, the heterogeneity of CS populations has led to varying clinical outcomes due, in part, to different responses to standard treatment modalities.3,4
Current algorithmic initiatives endorsing management based on hemodynamic profiling among patients with CS have predominantly focused on acute MI (AMI)-related CS.5 Recently, contemporary registry data have identified CS related to heart failure (HF), post-cardiotomy, pulmonary embolism (PE), or valvular heart disease as distinct clinical phenotypes to AMI-CS, with specific risk-related classifications and invasive hemodynamic profiles beyond the previously mentioned standard clinical definition.6 Within the AMI-, HF-, PE-, and VHD-related phenotypes, there are additional specific considerations, such as right ventricular infarction and fulminant viral myocarditis, refractory cardiac arrest (CA), PE burden, or stenotic or regurgitant valvular lesions, which have distinct invasive hemodynamic and imaging characteristics and unique modes of treatment with acute mechanical circulatory support (AMCS).7–9
CS can rapidly lead to the development of hypoperfusion (lactate elevation) and end-organ dysfunction (lung, renal, and/or hepatic injury), transforming a predictable hemodynamic event into a potential high-resource, intense, hemometabolic clinical catastrophe, which may be further challenged by its heterogeneous and time-sensitive nature.10–12 Although the use of AMCS continues to increase, there is a paucity of data on the management trends and strategies derived from the utilization of invasive hemodynamic monitoring.13 Importantly, distinct interpretations as to the value of a pulmonary artery catheter (PAC) arise from the extrapolation of studies among patients with acute decompensated HF without CS.14 Recently, the use of complete hemodynamics among patients with PAC for AMI- and HF-related CS was associated with increased survival.15 However, granular details on the information derived from the PAC and echocardiography as a tool to guide management remain limited among patient cohorts of AMI- and non-AMI-CS.16
Defining hemodynamic time zero in non-AMI-CS (compared to AMI-CS-derived metrics such as door-to-balloon time) and how early intervention and transitions affect the longitudinal outcomes of patients remain major global challenges.17,18 The administrative mechanisms and networks required to allow patients to transition to a center capable of providing timely clinical, hemodynamic, and imaging profiling in CS are limited; however, despite heterogeneous practices among healthcare systems, a well-outlined spectral severity of illness has been identified with novel severity-based classifications.19,20 Furthermore, the effect of delaying access to dedicated cardiac intensive care units with a hemodynamic-based approach in CS remains a frontier that affects our interpretation of transitions, disease trajectory, and severity staging while impacting our connection with frontline caregivers.21,22 Based on the scalable heterogeneity from a cellular level to healthcare systems in hemodynamic-based management of patients experiencing CS, we present considerations towards systematic hemodynamic-based transitions in which distinct clinical entities (AMI-CS, HF-CS, CA-CS, post-cardiotomy shock [PCS], PE-CS, and VHD-CS; Supplementary Material Table 1) share the common path of early identification and rapid transitions through an adaptive longitudinal situational awareness model of care that influences specific management considerations.23
Early Hemodynamic-based Recognition of Cardiogenic Shock
The proposal for the standardized care of patients with CS has been predominantly derived from an AMI-oriented perspective, driven by the need for time-sensitive therapeutic interventions such as revascularization therapy or AMCS to optimize door-to-balloon or door-to-unloading time, respectively.24,25 Despite the prior and ongoing randomization of patients on AMI-CS in focused randomized control trials (RCTs), there is a paucity of data leading to the identification of CS beyond clinical signs of hypotension and hypoperfusion.26 Furthermore, the inclusion of additional factors, such as heart and vascular mechanical complications, CA, extracardiac end-organ support (mechanical ventilation and renal replacement therapy), and underlying systemic or cardiac illness, has been poorly characterized.26 Severity-of-illness characterization remains limited by the early integration of dynamic clinical, hemodynamic, and imaging data in a systematic approach across the CS care spectrum.27,28
Data regarding comprehensive non-invasive (e.g. point-of-care ultrasound [POCUS], pulse oximetry index derived), minimally invasive (e.g. arterial pressure waveform derived), and invasive (e.g. PAC) hemodynamic monitoring for the diagnosis of CS are limited.29–31 Clinical information can be derived from a first responder evaluation of vital signs (e.g. tachycardia, pulse pressure), an electrocardiogram (e.g. injury, atrial or ventricular arrhythmias, conduction disease, and/or dyssynchrony), a chest X-ray (e.g. congestion, consolidation, mediastinal widening, cardiomegaly), and laboratory evaluation of end-organ function parameters (e.g. cardiac injury/congestion biomarkers, renal and hepatic function surrogates, and systemic inflammatory response syndrome responsiveness).32 POCUS imaging can estimate right and left heart function and rule out pericardial effusion and other AMI-related complications, such as acute mitral regurgitation (MR), ventricular septal defect (VSD), and free wall rupture.33
Among HF-CS and mixed phenotypes associated with myocardial injury, particularly in cases presenting de novo, myocardial function and structure can, for example, help identify fulminant myocarditis or amyloid heart disease diagnoses.34,35 Echocardiography can also provide comprehensive hemodynamics with quantitative measures of the derived cardiac index using the left ventricular outflow tract (LVOT) velocity time integral (VTI), and surrogates of estimated left and right heart loading conditions, including estimated right atrial pressure (RAP), pulmonary artery systolic pressure, and elevated pulmonary artery capillary wedge pressure (PCWP).36,37 Among those with a clinical and/or echocardiographic suspicion of CS, invasive hemodynamic evaluation can help with further CS profiling.38
Early Hemodynamic-based Transitions in Heart Failure-related Cardiogenic Shock
Once clinical and non-invasive hemodynamic and imaging evaluation has been established by first responders, patients with suspected CS should have immediate access to invasive hemodynamic, as well as valvular and coronary, profiling in the hybrid cardiac catheterization laboratory with percutaneous and surgical capacities.5 This facilitates a better understanding of cardiopulmonary interactions, particularly if patients have a high inotrope/vasoactive index and a need for additional respiratory and metabolic support with mechanical ventilation and/or renal replacement therapy.39 Furthermore, this allows for best practices of ultrasound- or fluoroscopic-guided access techniques and supra- or sub-diaphragmatic insertion of AMCS with subsequent distal limb protection.40,41 Patients with AMI-CS or PE-CS are distinct from other CS entities because, for example, coronary or pulmonary artery revascularization is a time-sensitive intervention that can affect left and/or right myocardial performance and subsequent disease trajectory.42,43 Patients in whom hemodynamic CS profiles indicate right heart-predominant congestion in the form of impaired RAP and pulmonary artery pulsatility index, even in the absence of hemometabolic CS and advanced Society for Coronary Angiography and Intervention stages, remain a high-risk cohort.44,45
Establishing invasive hemodynamic monitoring for both decision making and subsequent critical care surveillance (Figure 1) enables an ongoing conversation with the interdisciplinary team focused on:
- active management with hemocompatibility assessment;
- the choice and titration of inotrope/vasoactive agents;
- the choice of AMCS and evaluation of device dependency;
- consideration for revascularization, thrombectomy, or valvular interventions;
- cardiovascular-focused optimization of mechanical ventilation parameters;
- the addition of pulmonary vasodilators; and
- decongestion with diuretics and/or renal replacement therapy.46–49
Triggers for early hemodynamic re-profiling include: refractory hypotension and/or hypoxemia, intractable or worsening arrhythmias, major bleeding, right heart failure, structural or mechanical heart lesions, and performance limitations of AMCS.50 Index and follow-up invasive hemodynamic profiling is of critical importance among patients with an inability to recover and requiring evaluation for replacement therapies, such as durable ventricular assist device and heart transplantation.51
Multimodality Hemodynamic Management in Heart Failure-related Cardiogenic Shock
The clinical adaptation of hemodynamics in CS to evaluate responsiveness to cardiovascular therapeutics, including AMCS, remains a concept with limited data and multiple practice patterns across the globe.52 There is also limited information on the longitudinal impact of serial invasive PAC hemodynamic monitoring among patients with AMI-CS and HF-CS, and of routine pressure–volume loops to best understand dynamic ventricular–arterial coupling.53 The value of increased team-based awareness of impaired loading conditions, right and left heart unit axis coupling, and myocardial performance in CS incorporating imaging and hemodynamics remains elusive, with studies restricted to single-center cohorts with distinct approaches.54–56 However, the recently endorsed use of the PAC along with other device-associated parameters can help understand performance and trends in heart function and structure, as well as AMCS, including counterpulsation, transvalvular pumps, and/or extracorporeal membrane oxygenation (ECMO).57,58
Patients supported with counterpulsation with significant coronary disease, left heart congestion, and/or conditions associated with poor atrial compliance or severe MR can benefit from hemodynamic and imaging surveillance with dynamic sequential weaning and hemodynamic monitoring.59,60 Patients with transvalvular pumps also have central aortic pressure recording and pump positioning, and new-generation Smart Assist is able to provide additional information, such as surrogates of left or right heart loading conditions, including left or right ventricular end-diastolic pressure and cardiac output.61,62 Device-related surrogates can be then incorporated to available clinical, hemodynamic, and imaging information to best understand transitions to weaning and recovery.63
The role of invasive hemodynamics on veno-arterial ECMO (VA-ECMO) with or without transvalvular pumps or counterpulsation remains elusive.64,65 Importantly, invasive hemodynamics on all patients under AMCS can help with the early detection of complications related to hypovolemia associated with bleeding (low RAP), acute right heart failure (high RAP, elevated RAP/PCWP ratio and low right ventricular stroke work index), and cardiac tamponade related or not to specific AMI-related mechanical complications (elevated RAP and pulmonary artery diastolic pressure with associated hypotension).66 Furthermore, AMCS management (Figure 2) can be established by hybrid methodologies in which surrogates or right heart loading conditions can be identified non-invasively by echocardiography and left heart loading conditions can be identified among those patients with pre-existing invasive hemodynamic surveillance strategies (e.g. implantable pulmonary artery monitor) coupled with the above-mentioned clinical and routine echocardiography and device-related surveillance mechanisms.67,68
Although invasive hemodynamic monitoring and AMCS offer targeted guidance for the management of patients with CS resulting from different etiologies and provide information on the concomitant presence of other shock states (e.g. septic, hemorrhagic, distributive, or neurogenic), it is still critical to weigh the risk–benefit ratio of these diagnostic and therapeutic interventions in these critically ill patients. Whether percutaneous or surgical interventions, all require vascular access and put the patient at higher risk of complications, including vascular injury leading to excessive bleeding, thrombosis, or distal limb ischemia; myocardial injury leading to tamponade or valvular regurgitation; neurologic injury; and infection.69 In general, vascular sheaths range in size from 7 to 29 Fr, with larger sheaths facilitating access for the AMCS and providing more support.69
Furthermore, although hemostasis can be achieved by manual compression for those AMCS using smaller-sized vascular sheaths, closure systems such as the Angioseal, Perclose, or large bore closure devices can facilitate hemostasis after removal of AMCS using larger-sized vascular sheaths.69 Surgical closure may be required in the event of vascular injury. When using closure systems, it is important to understand whether the clinical trajectory of a patient may require vascular access at that site in the near term (i.e. within 3 months).

Hemodynamic Considerations in Mechanical Complications Related to AMI-CS
Although rare due to contemporary early reperfusion strategies, patients with AMI-CS can still present with mechanical complications (e.g. left ventricle [LV] free-wall rupture, VSD, papillary muscle rupture with severe MR, dynamic LVOT obstruction, pseudoaneurysm, and true aneurysm) leading to acute pulmonary edema or refractory CS.70,71 Hemodynamic monitoring can elucidate the consequences of such complications, whereas bedside echocardiography or POCUS can further identify their type, size, and location, along with the presence and direction of shunts. Initial resuscitation efforts may require vasoactive drugs and mechanical ventilatory support.70,71
Positive-pressure ventilation can improve gas exchange and hemodynamics by augmenting cardiac output by reducing MR and LV afterload.70,71 AMCS is often required for additional myocardial unloading and hemodynamic stabilization, particularly as a temporary bridge to definitive revascularization and delayed myocardial rupture or aneurysm repair to facilitate reduced patch dehiscence and postoperative bleeding from antiplatelet therapy.70,71 In the perioperative setting, AMCS can help attenuate respiratory and hemodynamic deterioration during anesthetic induction for intubation.70,71
Although surgery has long been the mainstay, percutaneous options in patients with prohibitive surgical risk are emerging.71,72 Notably, most studies evaluating AMCS in CS largely excluded patients with mechanical complications (e.g. the IABP-Shock II trial and SHOCK Trial registry).4,42,71 Patients with multiorgan failure may benefit from biventricular mechanical circulatory support or VA-ECMO with LV venting. In these instances, the LV vent reduces LV afterload, the pulmonary shunt fraction, and pulmonary edema; this facilitates improved gas exchange, reduces acute lung injury and Harlequin or North–South syndrome, and minimizes the risk of LV or aortic thromboses.70,71 In VSD, certain venting strategies, such as the Impella, may exacerbate right-to-left aspiration of deoxygenated blood and peripheral systemic embolization of necrotic LV debris.70,71
Hemodynamic Considerations in Cardiogenic Shock After Cardiac Arrest
The ARREST trial recently showed that ECMO-facilitated resuscitation increases survival compared with standard advanced cardiac life support protocols in patients presenting with out-of-hospital CA and refractory ventricular fibrillation or tachycardia.73 The trial was stopped early given the significant survival benefit achieved with early ECMO. The criteria used in the trial included shockable rhythm, no return of spontaneous circulation after three defibrillation shocks and an estimated transfer time of <30 minutes.73 Patients presenting with two or more of these criteria have an extremely poor prognosis. Time from the 911 call to ECMO initiation has been shown to be the most important independent predictor of survival in these patients.73
The use of PAC for hemodynamic profiling is recommended in all patients undergoing AMCS, including ECMO therapy, to monitor effectiveness, determine the need for escalation, optimize device settings, and guide the timing and rate of weaning.15 Furthermore, hemodynamic practices used by VA-ECMO centers around the world highlight some areas that need continuing evaluation, including choosing the ideal cannulation strategy for each patient, setting the flows and monitoring the interaction of the circuit and the patient’s circulation, dealing with LV pressure and volume overload, and removing support either after recovery or for palliation purposes. Echocardiography provides many advantages in these scenarios: it may help direct therapy by defining the exact pathologic process leading to CS; it allows real-time guidance of wires and cannulas and reassessment of cardiac performance during cannulation; and it is crucial when weaning VA-ECMO because parameters such as LV ejection fraction (LVEF) >20–25%, LVOT VTI >12, the absence of LV dilatation, and no cardiac tamponade have been suggested to indicate successful weaning.74
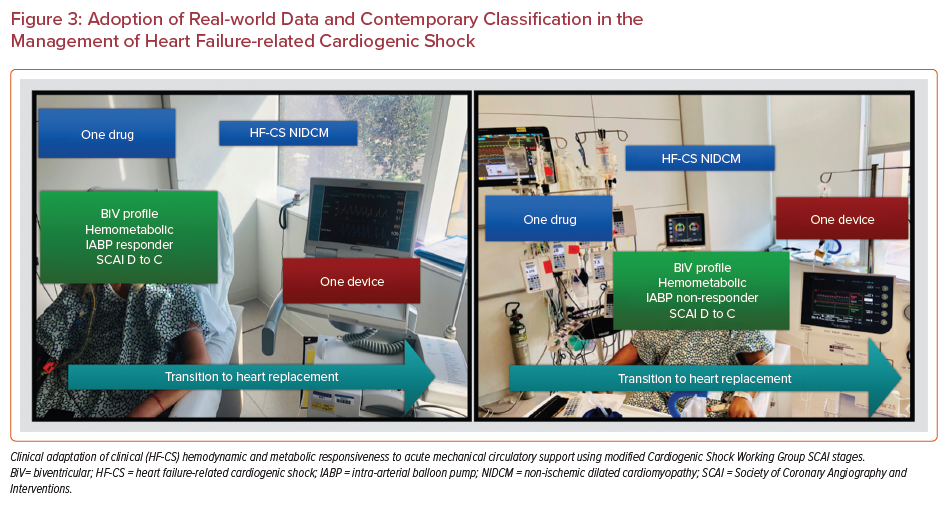
Numerous adjunctive LV unloading strategies have been implemented to decompress the LV mechanical load. LV afterload is increased after initiation of VA-ECMO, which can lead to progressive LV distention and stasis in the setting of extremely poor cardiac function. This can exacerbate arrhythmias, myocardial ischemia, pulmonary edema, thrombus formation, and myocardial recovery. A systematic review and meta-analysis explored the efficacy, safety, and optimal timing of LV venting in patients with CS requiring VA-ECMO.75 Most patients in the study had LV venting with an intra-aortic balloon pump and more than 50% of patients underwent early venting (within 12 hours). The study concluded that LV venting significantly improved weaning from VA-ECMO and decreased short-term mortality, especially if implemented early.75 There are currently two ongoing randomized trials, namely Early LA venting during venoarterial ECMO support (EVOLVE-ECMO; NCT03740711) and the Impella CP with VA ECMO for Cardiogenic Shock (REVERSE; NCT03431467), which may provide alternative options for LV venting.
Various studies have described predictors of successful weaning from VA-ECMO. In 2010, Aissaoui et al. described a weaning strategy that tested daily hemodynamic tolerance of ECMO flow reduction trials using clinical, hemodynamic, and Doppler echocardiography parameters associated with successful ECMO removal.76 All weaned patients had partially or fully recovered severe cardiac dysfunction, had tolerated a full ECMO weaning trial, and exhibited certain echocardiography parameters (VTI ≥10 cm, LVEF >20–25%, and spectral tissue Doppler imaging mitral annulus peak systolic velocity ≥6 cm/s at minimal ECMO flow support).76 Currently, weaning strategies are mostly based on echocardiography parameters, as highlighted above. However, these parameters fail to provide information about right heart function, systemic hemodynamics, and tissue perfusion. Success of resuscitation from circulatory shock involves normalization of microcirculatory and tissue perfusion. Impairment of sublingual microcirculation has been associated with CS, and inability of VA-ECMO to recruit this microcirculatory alteration predicts adverse outcomes following its implementation.
The results of a prospective observational study by Akin et al. showed that functional parameters of microcirculation, including total vessel density and perfused vessel density, reflect recovery from CS and predict successful weaning from VA-ECMO.77 Many areas of uncertainty remain, and the role and application of extracorporeal cardiopulmonary resuscitation (ECPR) remain controversial. For example, the definition of ECPR lacks granularity, and there are no clear inclusion or exclusion criteria to determine which patients would benefit from the procedure. Finally, the most commonly considered outcomes of ECPR are death, neurological damage, and multiorgan failure. However, other possible outcomes should be considered. For example, in patients evolving to brain death, ECPR ensures end-organ perfusion, making them suitable for organ donation. In addition, patients who do not recover appropriate cardiac function but remain neurologically intact can be further bridged to heart transplant or durable mechanical circulatory support.
Conclusion
CS remains a challenging entity affecting patients and health systems across the globe, requiring an interdisciplinary system-wide approach to avoid hemometabolic presentation and to foster an environment of early transition to remission, replacement, and/or palliation. Hemodynamic-based management of CS within a platform of situational awareness can help advance the care pathways among prehospital and front line caregivers. Further research is needed in order to best acquire, scale, and transparently present and understand longitudinal transitions using hemodynamic surrogates at different levels of care and across distinct clinical phenotypes within a wide spectrum of illness and therapeutic options. Creating longitudinal registries with granular systematic data adjudication can help deconstruct this major global public health problem by triggering quality and patient-reported benchmarks in addition to adequate design of RCTs across a network of CS centers. Finally, future studies also need to explore timing around initiation and weaning, the optimal management of drugs and devices, and the engagement of systems of care for patients with CS. Although RCTs can provide the highest level of evidence, observational and registry-based analyses of real-world experiences (Figure 3) may help identify how to design such studies and which clinical care gaps need to be explored.